For efficient processes
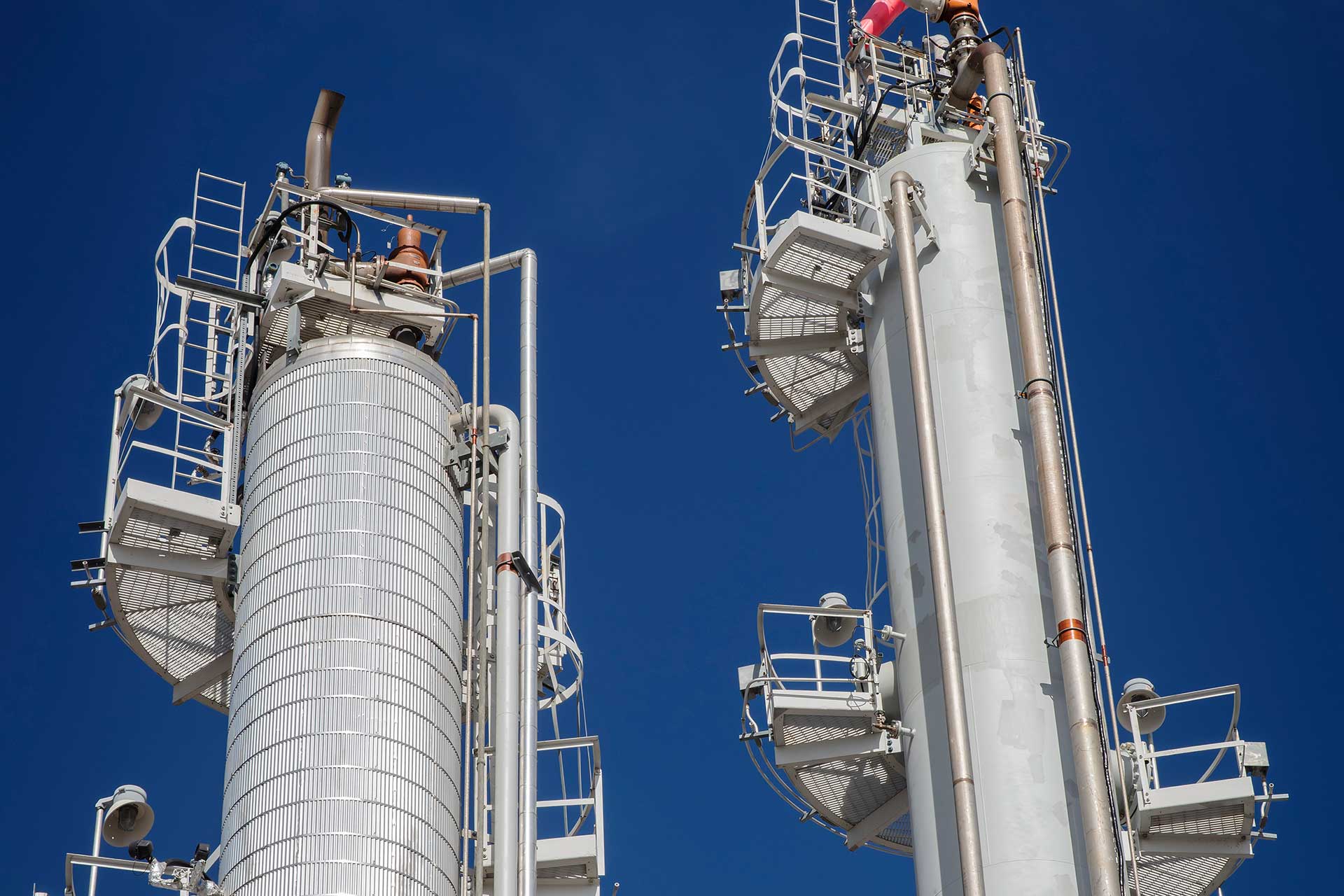
For process intensification purposes, pervaporation membrane/membrane separation technology can very well be integrated into other chemical (separation) processes: smart combinations with compact equipment for efficient processes. Examples are the breaking of azeotropes and in-situ dehydration during reactions.